Abstract
Over 60% of the original oil in a place (OOIP) is retained in a reservoir after conventional methods have been exploited. Application of enhanced oil recovery (EOR) technology gives an additional chance to get out possibly about 20% more oil from the reservoir. The use of water-soluble polymers improves the water–oil mobility ratio, therefore, the displacement efficiency increased, and leads to enhanced oil recovery. High-molecular-weight polyacrylamide group is widely and successfully used in EOR. But no commercial polymer composition can be used in conditions of high temperature and hardness brine offshore reservoirs yet. To avoid the time consumption and high expense for selection and synthesis of the appropriate-structural polymer for EOR application, we attempt to find additives to enhance the thermal stability of polymer solutions. In this paper, we report the results of improved viscosity stability of diluted polymer/seawater solutions aged at reservoir conditions for 31days by adding graphite-oxide particles (GOs). In the presence of 300 ppm of GOs, the viscosity stability of 1700 ppm acrylamide-based polymer in sea water solution increases from 92 °C to 135 °C. FESEM pictures show good distribution of GOs in polymer network, which is a result of integration of functional groups in GOs surfaces and hydrophilic polymer chains.
Export citation and abstract BibTeX RIS

Original content from this work may be used under the terms of the Creative Commons Attribution 3.0 licence. Any further distribution of this work must maintain attribution to the author(s) and the title of the work, journal citation and DOI.
1. Introduction
Over 60% of the original oil in a place (OOIP) is retained in a reservoir after conventional methods have been exploited. Application of enhanced oil recovery (EOR) technology gives an additional chance to get out possibly about 20% more oil from the reservoir [1]. The use of water-soluble polymers improves the water–oil mobility ratio, therefore the displacement efficiency increased and leads to enhanced oil recovery. High-molecular-weight polyacrylamide (AM) group is widely and successfully used in EOR. However, given the harsh conditions present in most oil reservoirs, new problems and limitations arise with the use of water-soluble polymers. Besides, positively affecting solution rheology, water-soluble polymers should withstand high salt concentration, the presence of calcium, high temperatures (>70 °C) and long injection times (at least 12 months) [2]. High salt concentrations reduce the thickening capability of most ionic water-soluble polymers, while the presence of calcium leads to flocculation [3]. Mobility control and sweep efficiency is achieved only if viscosity is maintained during polymer propagation. There are four main types of degradations affecting the viscosity of a solution containing acrylamide based polymer: thermal, free radical, mechanical and biological. We will focus on the two first types of degradation grouped under the name chemical degradation.
1.1. Polyacrylamide: chemical degradation and its prevention in reservoir conditions
1.1.1. Thermal degradation
Thermal degradation of polyacrylamide is related to chemical change of the functional groups of the polymer. In thermal environment, the hydrolysis of acrylamide moieties occurs. New anionic charges are generated on the backbone chain of the polymer. In the presence of divalent ions (Ca2+, Mg2+), the viscosity of polymer solution will be reduced due to bridging effect of Ca2+, Mg2+ with anionic charges of the polymer, and precipitation may happen partially [4]. The rate of hydrolysis is dependent on the temperature: the higher the temperature, the higher the rate. To reduce the negative effects of reservoir temperature and hardness, the polyacrylamide can be modified with functional monomers, such as N-vinyl pyrrolidone (NVP), or sodium acrylamido tertbutyl sulfonate (ATBS) to improve the tolerance to divalent contained brine and to protection to hydrolysis, thanks to the neighboring effect [5]. Nevertheless, ATBS has some disadvantages because hydrolysis can occur by itself as well. NVP can protect polyacrylamide from hydrolysis better [6] but due to its low reactivity in polymerization, it is difficult to achieve the targeted viscosity for polymer flooding.
1.1.2. Radical degradation
Radical degradation is related to the generation of radicals that can react with polyacrylamide backbone chairs resulting in the reduction of molecular weight [7] and the decrease of viscosity of the polymer solution due to the reduction of hydrodynamic volume. These radicals can be generated by heat through the cleavage of weak links in the polymer chains, impurities in polymer chains during processing etc. Free radicals can be generated by the presence of oxygen impurities in the environment. The presence of Fe2+ or/and H2S is also known to induce an acceleration of radical formation in the presence of oxygen (redox system).
Beside synthesis of specific copolymers with monomers NVP and ATBS as indicated above, there are some solution and protective additives used to increase chemical and thermal stability of polyacrylamide: Muller [8] has shown that the impurities of polymer products can be removed if purification with methanol is applied in the production process. Nevertheless, no producer has done it yet because it is not beneficial and economical, and environmentally friendly.
1.1.3. Oxygen removal
Seright and Campbell have shown that removing dissolved oxygen to a level below 5 ppb would allow solution of polyacrylamide to keep viscosity stable at 120 °C for more than 200 days [9]. But it will be impossible at macroscopic scale because of leakage, and as soon as the dissolved oxygen level reaches above 30 ppb, acceleration of the viscosity loss is observed. As the effective oxygen scavengers and reducing agents, dithionite and sulfite can reduce dissolved oxygen to a level of 50 ppb to 500 ppb. Below that, a new resource of oxygen appears because of very active redox system.
1.1.4. Radical scavenger and sacrificial agent
The idea of using sacrificial agent to eliminate viscosity drop of anionic polymer solution has attracted many studies. Generally, alcohols having labile hydrogen are often used as a sacrificial agent to prevent radical degradation of polymer backbone. Radical scavengers may have a better effect on elimination of polymer radical degradation due to the possibility of giving a proton to trap the radical species to form a new stable radical. Some examples of effective radical scavengers such as phenylphosphonic acid, thiourea, sodium thiocyanate, mercaptobenzo family are known [10]. There is a synergistic effect between sacrificial agent and radical scavenger like isopropanol (IPA) and thiourea. As was reported, the combination of IPA and thiourea becomes one of the best stable mixtures to prevent polymer from radical degradation [11].
1.1.5. Complexing agent, chelating agent
To eliminate the effect of degradation of the polymer backbone due to the presence of iron, the complexing agent can be used. Ethylene diamine tetra acetic acid (EDTA), sodium citrate, sodium azide etc, have been reported as effective compounds in polymer degradation reducing [12]. Nevertheless, using the chelating agent may easily change the pH in reservoir fluids that leads to unpredictable reaction in long-term production life. One more question is that it will require additive chemicals which often negatively affect economical impact of the methods.
1.2. Graphene oxide and its role in EOR application
Production of graphene oxide (GO) via oxidization of graphite with strong oxidizing agents (a combination of KMnO4, KClO4, NaNO3 and H2SO4) has been known as Hummer's method since 1956 [13] but was then forgotten for a long time. Only after the discovery of graphene and the resulting Nobel Prize in Physics in 2012 did GO receive widespread attention as an intermediate material in the fabrication of graphene [14–16]. GO is a compound made up of a carbon skeleton with main functional groups, such as carboxyl, carbonyl, epoxy and ether groups and hydroxy groups. These functional groups enable chemical reactions of GO [17] to form covalent bonds with other compounds (e.g. esterification, amidation). Another option is a GO reaction to form non-covalent bonds. The possible types of the bonds are hydrogen bonds, van der Waals forces, H-π, cation-π, anion-π, π–π, electrostatic forces. These non-covalent bonds are employed in preparation of composite polymers, biopolymers and in using adsorption and absorption properties of GO.
GO is widely studied for use in oil and gas production and exploitation (oil and gas P&E) but mainly as a promising additive for fluid-loss control in advanced drilling fluid [18]. Another work has been patented [19] related to the use of GO as a viscosity enhancer for EOR. But this idea until now has not been realized anywhere. McCoy et al [20] have published results on the possibility of GO in stabilizing crude oil in water emulsion.
Our research was based on the idea that if GO is an excellent filler of composite polymers due to its reaction to form non-covalent bonds, can we use GO for enhancing properties of polymer solution in brine for EOR in high temperature (135 °C) offshore reservoirs such as the Oligocene at White Tiger Oilfield (WT) in Vietnam?
2. Experimental
2.1. Employed chemicals
Graphite PM is very fine crystalline powder graphite, mesh 0.025 mm (Merck Chemicals).
Sulfuric acid, nitric acid, potassium permanganate, thiourea are from Merck Chemicals.
Anionic acrylamide based polymers: copolymer of AM and ATBS flopaam AN125 (molecular weight 6.106 g mol−1, recommended for reservoir temperatures up to 95 °C) and terpolymer of AM, ATBS and NVP superpusher SAV 225 (suitable for reservoirs with temperature as high as 120 °C) were obtained from SNF FLOERGER. Sea water was obtained from Oligocene mine, White Tiger Oilfield (WT), Vietsovpetro JV (VSP) with total salinity of 36.6% and hardness of 2118 mg l−1.
2.2. Preparation of graphene oxide and methylated graphene oxide
Some modifications were made to the Hummers method [13] as described in [14, 21] applied to the preparation of graphene oxide (GO) from graphite PM. In a typical reaction, potassium permanganate (15 g) and graphite (5 g) were initially stirred until becoming a homogeneous mixture. Then, in a round-bottom flask (500 mL) with ice-water bath, concentrated sulfuric acid (98%, 100 mL) was added to the mixture with continuous stirring until a uniform liquid paste was formed. The water bath was removed. The stirring continued until a foam-like intermediate spontaneously formed (around 30 min) at room temperature with a large volumetric expansion. Deionized water (400 mL) was added, and rapid stirring was restarted to prevent effervescing. Next, the flask was placed in a 90 °C water bath, and after 1 h a dark yellow homogeneous suspension was obtained. The suspension was then filtered and was subjected to repeated washing by deionized water (200 ml), 30% HCl solution, then two times ethanol using centrifugation (6000 rpm) until all Cl− ions were removed. The final product was dried in vacuum at room temperature.
Methylation of graphene oxide by methanol: after the above-presented steps, 300 ml of methanol was added and stirred for 10 min, then again centrifuged and washed three times with methanol. The obtained solids were dispersed in 300 ml of methanol and 10 g of H2SO4. The reaction mixture was stirred and circulated for 5 h, cooled, then rinsed five times with 150 ml of methanol to obtain completely clean acid before being dried to obtain the product.
2.3. Preparation of polymer solutions
A polymer solution was prepared by using magnetically driven stirrer. The polymer was added slowly on the shoulder of the vortex formed by brine to avoid formation of slub. As soon as the entire polymer was added, the rotor speed was reduced from 300 to 80 rpm to avoid mechanical degradation. Stirring was turned off after 3 h and the solution was kept at room temperature for the next 48 h for complete hydration.
2.4. Evaluation on thermal and rheological stability of polymer solutions at 135 °C
The studied samples with certain polymer/additive concentrations dissolved in WT brine were added into the ace glass heat-resistant ampoules, deoxygenated with nitrogen and tightly close.
- Put ampoules in the oven and incubate attemperature of 135 °C.
- Observe the transparency of annealing solutions at the start and after 7, 14, 21 and 28 days.
- Measure viscosity of solutions at the start and after 7, 14, 21 and 28 days, and estimate viscosity loss.
2.5. Measurement instruments
Graphene oxide (GO) and methylated graphene oxide (GOM) samples were characterized using various analytical methods such as optical Fourier transform infrared spectroscopy (FTIR) on Bruker Equinox 55 devices, field emission scanning electron microscopy (FESEM, Hitachi S-4800), x-ray diffraction (XRD) using Bruker D8 Advance, and thermogravimetric analysis (TGA, TG/DTA) on STA-PT 1600. Viscosity of solutions was measured using Viscometer Brookfield DVIII+.
3. Results and discussion
3.1. Graphene oxide and methylated graphene oxide characterization
There are a number of publications refereeing to the preparation and characterization of graphene oxide (GO) and methylated graphene oxide (GOM). Our obtained data were almost no different from what we were looking for. Due to our focus being application in an oilfield, it is very important to see how those additives can be dispersed in high salinity brine.
As we can see from figure 1, 400 ppm of GO can be dispersed well in high salinity WT brine. With concentration more than 400 ppm, some light clusters were observed during the aging process but they are easily dispersed again after small vibration.
Figure 1. GO dispersion in brine. (a) 200 ppm of GO in brine, (b) 300 ppm of GO in brine and (c) 400 ppm of GO in brine.
Download figure:
Standard image High-resolution image3.2. GO and GOM interaction with diluted polymer solutions and possibility of enhanced thermal stability
In the proposed structure of GO and GOM particles in figures 2 and 3, we can see in the carbon skeleton numerous functional groups, such as carboxyl (and its methylated in GOM), carbonyl, epoxy and ether groups and hydroxyl groups, as a result of deep oxidation of graphite. These functional groups make GO and GOM become more hydrophilic and better dispersed in electrolyte solutions such as brine than their graphite precursors. When water-soluble polymer is disoved in brine, due to hydrophilic similarity and small size (≈100 nm), GO and GOM particles can easily interfere with interlocking polymer backbone chains, making more durable solution. In high-temperature environment, when polymer degradation occurs, the functional groups in GO and GOM can react and particles can play a role as a reducing and sacrified agent, leading to slowing down or limiting the degradation process.
Figure 2. GO structure.
Download figure:
Standard image High-resolution imageFigure 3. GOM structure.
Download figure:
Standard image High-resolution image3.3. Evaluation of the thermal stability of polymer solution in aging process at 135 °C
The viscosity changes in polymer fluid samples during aging process at 135 °C. Viscosity loss (%) can be calculated by the following formula with the viscosity of brine as a basic line:
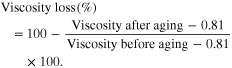
From the results in table 1 and figure 4, we can see that with 300 ppm of GO added into solution of 1700 ppm polymer AN 125, the thermal stability increased from 92 °C to 135 °C with the viscosity loss after 28 day aging being about 53.7%. This composition has shown the viscosity stability much better than what we get with SAV-a very expensive tert-polymer of AM, ATBS and NPV.
Table 1. The viscosity changes in polymer fluid samples during aging process at 135 °C.
Viscosity (cP) | |||||||
---|---|---|---|---|---|---|---|
No | Sample contain (ppm) | start | 7 days | 14 days | 21 days | 28 days | Viscosity loss (%) |
1 | AN125 2000 (AN2000) | 1.28 | 1.14 | 1.02 | 0.88 | 0.78 | 100 |
2 | AN125 1700 + GO 300 (AN17 GO3) | 1.22 | 1.16 | 1.12 | 1.11 | 1.00 | 53.7 |
3 | AN125 1700 + GOM 300(AN17 GOM3) | 1.65 | 1.11 | 0.86 | 0.82 | 0.80 | 100 |
4 | AN125 1700 + Thiourea300 (AN17 U3) | 2.63 | 1.48 | 1.23 | 1.22 | 1.14 | 60.8 |
5 | SAV 2000 | 2.45 | 1.18 | 1.01 | 0.92 | 0.75 | 100 |
6 | SAV 1700 + GO300 (SAV17 GO3) | 1.91 | 1.41 | 1.32 | 1.31 | 0.98 | 85.5 |
7 | SAV 1700 + GOM300 (SAV17 GOM3) | 2.22 | 1.24 | 1.09 | 1.04 | 1.02 | 84.4 |
8 | SAV 1700 + Thiourea300 (SAV17 U3) | 2.33 | 1.49 | 1.45 | 1.38 | 1.35 | 64.5 |
9 | Brine | 0.81 | 0.81 | 0.81 | 0.81 | 0.81 | 0 |
Figure 4. Graphs of log viscosity during the aging of polymers at a temperature of 135 °C.
Download figure:
Standard image High-resolution image3.4. Morphology study of the polymer/additive distribution in diluted brine solution by FE- SEM pictures
We can see in figure 5 that GO particles have a very porous structure, especially after aging. It can be proposed that all functional groups, mainly with acidity in GO, are easily exposed in brine environment and react with functional groups, mainly with anionic nature in AM-ATBS copolymer and the compatibility and synergistic effect can occur.
Figure 5. 300 ppm of GO in brine (a) before and (b) after aging.
Download figure:
Standard image High-resolution imageFigure 6 provides the basis for this proposal: before aging, the mixture of 1700 ppm of AN125 and 300 ppm of GO in brine looks shrinking, despite levels being relatively low. The morphology has sharply changed after aging, the polymer brush shows that all its needles and GO particles can easily be distributed among them. Perhaps this can explain the enhancement of viscosity loss of this mixture, comparing to the original AN125 itself.
Figure 6. AN125 1700 + GO300 in brine before and after aging.
Download figure:
Standard image High-resolution imageFigures 7 and 8 show a very porous structure of GOM and a needle form of SAV 225 diluted in brine. But it is difficult to explain why the mixture of SAV and GOM has quite high viscosity loss after aging. Perhaps the compatibility in this mixture was not as high as in the previous one due to less chemical activity of GO methylated form and NVP by itself. Figure 9 shows the structure of SAV 1700 + GOM300 in brine before and after aging.
Figure 7. 300 of GOM in brine.
Download figure:
Standard image High-resolution imageFigure 8. SAV 1700 in brine.
Download figure:
Standard image High-resolution imageFigure 9. SAV 1700 + GOM300 in brine (a) before and (b) after aging.
Download figure:
Standard image High-resolution imageFrom figures 10 and 11, it is difficult to say anything regarding the sample structure. The thiourea in the mixture with IPA is recognized as a best sacrificed agent for chemical degradation control in high temperature medium. But there are a number disadvantages working with IPA in the oilfield: flammable and high extra cost. For temperature as high as 135 °C, it may be that thiourea cannot have enough shielding effect to protect polymer solution from degradation.
Figure 10. SAV 1700 + thiourea300 in brine (a) before and (b) after aging.
Download figure:
Standard image High-resolution imageFigure 11. GOM200 + SAV1800 + thiourea300 (a) before and (b) after aging.
Download figure:
Standard image High-resolution image4. Conclusion
By adding 300 ppm of GO into the mixture of 1700 ppm co-polymer of AM and ATBS, we successfully enhanced its thermal stability from 92 °C to 135 °C while aging at Oligocene reservoir conditions in four weeks. Other well-known high temperature resistant polymers with/and sacrificed agent have much higher viscosity loss in the same screening conditions. This opens a great opportunity to use GO as a thermal stability additive for polymer solutions in polymer enhanced oil recovery with harsh conditions. Repeated evaluation and better thermodynamic study have to be carried out.
Acknowledgments
This work was partly financial supported from MOST: KC.02.DA.06/11-15 and from VAST: VAST.SXTN.02/14-15